“A natural or man-made system is said to be chaotic if its future is unpredictable and if it is extremely sensitive to initial conditions,” said Paul Woafo of the Department of Physics, at the University of Yaoundé I, Cameroon. “Different to the chaos due to stochastic interactions, deterministic chaos has special signatures. It has shown its presence in all scientific fields from natural sciences to humanities. Avoided in some systems, it is used for the improvement of some technological processes: cryptography, domestic appliances, electrical treatment of diseases such as heart arrhythmias and epilepsy.”
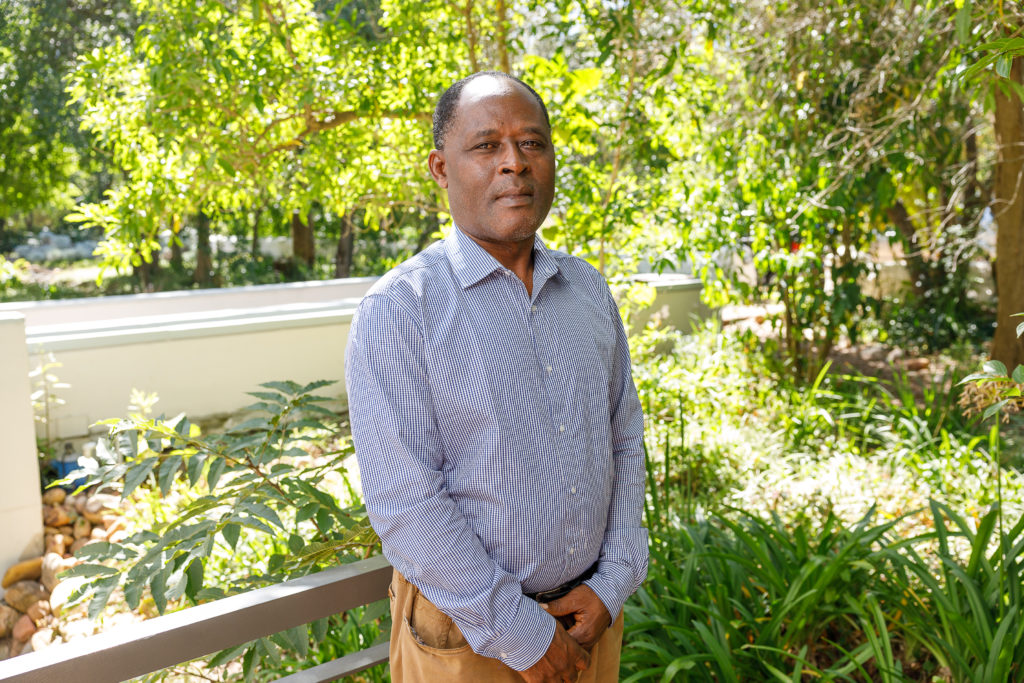
With this opening, Woafa took STIAS fellows on a fascinating journey through the world of omnipresent chaos and how chaos can be put to use productively.
He defined omnipresent chaos as follows: “a dynamical system is said to be chaotic when it is deterministic (without any disordered interactions) but its temporal behaviour is unpredictable and extremely sensitive to initial conditions.”
Woafo explained that chaos theory started with human attempts to understand the interactions of the three major masses that interact through gravitational forces in our world – the sun, earth and moon, and to answer questions about the stability of the solar system. “At the end of 19th century French mathematician and theoretical physicist Jules Henri Poincaré demonstrated that the trajectory of each body in a three-body system may evolve chaotically.”
“Recent simulations show that the planets’ trajectories in the whole solar system are chaotic so that it’s not possible to predict their actual position for a time range of 60 million years. Obviously, an impossible time period to verify,” he added.
Woafo also highlighted the work of then PhD student Yoshisuke Ueda in 1961 who found random or disordered behaviours in electronic systems – providing the first experimental evidence that chaotic phenomena were possible in electronic circuits. His work was, however, only published in 1970.
He also focused on the work of Edward Norton Lorenz who is regarded as the father of chaos theory for his work looking at complex meteorological spatiotemporal equations and observation that there was “no order in the data sequence over large time periods for some of the values of coefficients as well as sensitivity to initial conditions. This led to the first confirmation of chaos in computer simulations and is the most cited reference in chaos theory,” said Woafo.
After these first research works on chaos theory, scientists looked for chaos in other fields including systems described by discrete equations. Robert May studied the discrete logistic equation which describes the link between the density of the biological population to that of the next generation and showed a random variation of population density over time. “A birthrate greater than 3.57 shows chaos and it is no longer possible to determine the population density,” said Woafo. “This has subsequently been demonstrated by biologists.”
He also pointed to two important methods to prove the existence of chaos – including the Lyapunov exponent in mathematics that measures the trajectory divergence when there is a change in initial conditions and frequency spectrum analysis which involves studying the frequency spectrum components of a signal.
Studies and books published from the 1990s onwards made it clear that chaos is present in all fields – from biology to chemistry and biochemical systems, economics and finance, and the social sciences. “Now it’s said that chaos is everywhere,” said Woafo.
Predicting and manipulating chaos
Using the example of the changing seasons, Woafo showed how it’s possible to look at the time evolution of systems which allows us to predict when chaos is coming showing intermittent routes, period-doubling routes, quasi-periodic and sudden transition routes to chaos.
He also pointed two methods used to manipulate chaos – “Chaos-control methods have been developed using mathematical models, numerical simulation and experiment – this can change a chaotic state to a periodic state and back – in other words anti-control of chaos.”
“We can also synchronise various chaotic systems by coupling them together – in phases, delayed, generalised, anticipated and complete synchronisation,” he added.
The existence of chaos and the possibility of synchronising chaotic systems lead to some very interesting applications ranging from improving the energy efficiency of home appliances like mixers and sieves to chaos cryptography of images, text, audio and audiovisual. “At emission a message is encrypted with the first chaotic signal and sent to the transmission channel where another chaotic signal is synchronised to the one at emission to decrypt.”
“It’s also being used for human application,” continued Woafo. “Anti-control chaos is being tested in animal models for epilepsy therapy. Mathematical models predict the transitions between normal and epileptic activity – transitions from normal functioning (chaos) to synchronous oscillations (epilepsy). Applying a small electrical stimulus may be able to alter these transitions.”
Bio-inspired electronic oscillators
But, how can we also make natural chaos work for us? Woafo turned to work in his laboratory and elsewhere on bio-inspired electronic oscillators.
“Biological oscillators are at the centre of many processes in living bodies,” he explained. “They possess some typical features generating self-sustained oscillations with periods varying from a microsecond to a day, and include biological phenomena such as cardiac rhythms, breathing, neuronal firing, vasomotion, menstrual cycle and circadian regulation. They present special features including pulse-like behaviour, relaxation as well as chaotic signals and bursting.”
Mimicking these and being able to predict their behaviours, understand their functioning and develop mathematical equations for similar electronic circuits can lead to the development of bio-inspired products with a range of functions.
“The understanding of their working principles leads to the development of new electrical circuits used in electrical engineering,” said Woafo, “but also potentially to generate artificial medical devices (like pacemakers and heart-muscle simulators) or artificial toys/bio-inspired devices that mimic birds, insects, millipedes, fish, snakes, etc. that can be used in defence and security.”
Using these principles, Woafo’s group is currently working on a heart-pump device as well as a flying bird toy that can imitate different bird-flight modes. (Fellows were treated to a video of the ‘bird’ in action.) Woafo explained that such artificial birds could replace drones with spying and military uses as well as the ability to search in emergency rescue situations. “I predict these will be used generally in less than 10 years – if they are not being used already,” he said.
Michelle Galloway: Part-time media officer at STIAS
Photograph: Ignus Dreyer