“The advent of single-cell RNA-sequencing, a quantum leap in biotechnology developed through collective ingenuity, has propelled us into a new era of biology and biomedical research,” said Rickard Sandberg of the Department of Cell and Molecular Biology at the Karolinska Institute, Sweden. “For the first time, this powerful tool allows for the measurement of expressed genes across thousands of individual cells simultaneously, offering an unparalleled view of the roles of individual cells within the tissues of our body. Collectively generating an entire atlas of cell types in humans is currently in progress.”
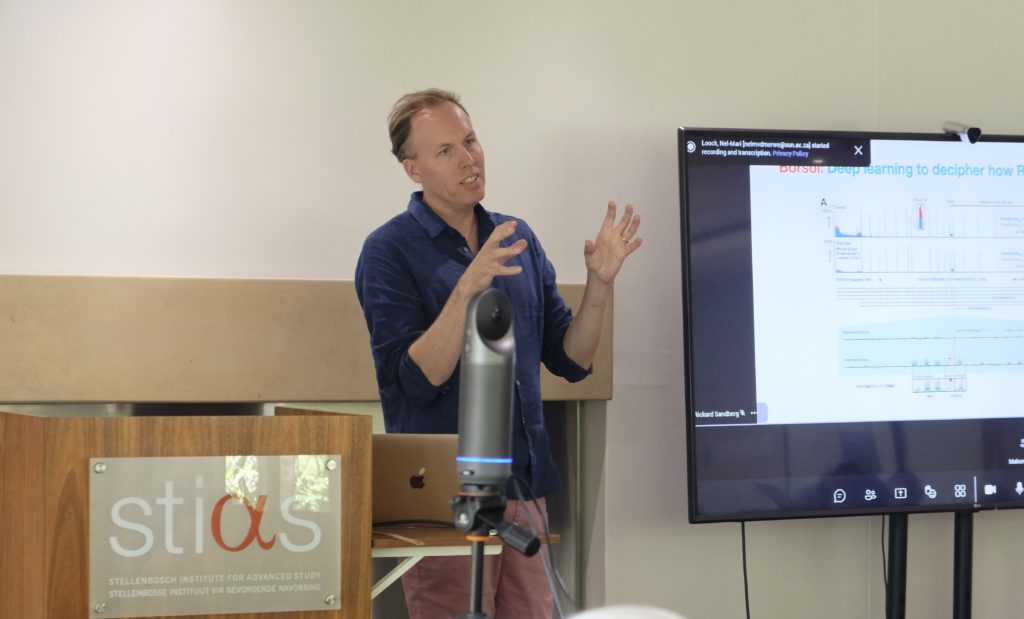
Sandberg is one of the pioneers in this area, and his laboratory has developed several core single-cell RNA-sequencing methods.
“This seminar is an invitation to learn about a milestone in molecular biology and to appreciate the nuanced choreography of gene expression that single-cell RNA-sequencing has revealed. I hope to deepen your appreciation for the cellular underpinnings of life.”
Diving into our cells
Starting with the basics, Sandberg described cells as the fundamental units of life – the building blocks of all organisms that orchestrate all biological functions. There are different types of cells with different functions but at the core of cellular identity lies the genome — each cell endowed with the same genetic information (a DNA code comprising four letters – ACGT) yet differentiated into a myriad of cell types by expressing (i.e. using) select subsets of genes.
“Our cells form a genetic library where the volumes are the chromosomes. Each volume contains 1000s of books each providing instructions for unique proteins. But the library also contains diligent librarians who are taking photocopies – messenger RNA (mRNA) – which informs cell function as it can be read by ribosomes and allows them to undertake the process of synthesising proteins,” explained Sandberg.
“Although all cells have the same genetic information,” he added. “The different functions come from using different parts of the gene library – turning genes on and off like a dimmer light.”
In 2000 US President Clinton announced the completion of the draft human-genome sequence – which comprised three billion letters (bases) and 20 000 unique protein-coding genes. A mammoth task requiring many years of work. Nowadays, of course, things happen much quicker and Sandberg pointed out that 1.6 billion sequences (200 letters each) can be sequenced in 48 hours at a cost of EURO 3000 – meaning we have a lot more powerful data at our disposal.
But, as Sandberg explained, when it comes to understanding cells we don’t just want an overview, we want to be able to count and understand the functioning of each chapter of the cell book.
“Cell-population analysis is like studying a smoothy while single-cell analysis is a like a fruit bowl containing individual fruits.”
“The ability to characterise cell types and their genetic programmes across tissues, has profoundly accelerated through the development of single-cell sequencing methods. These innovations in molecular methods, specifically over the last ten years, have enabled individual cells – of any organism – to be molecularly characterised,” he said.
And Sandberg and his colleagues have developed some of the most well-used single-cell RNA-sequencing methods (notably Smart-seq3xpress) which have been used extensively to study cell types in health and disease.
Using single-cell sequencing can identify 1000s of types of cells with significant gene-expression factors making it possible to dissect and illuminate the dynamic process of gene expression. For example, by sequencing the RNA molecules in a cell, researchers can directly obtain information on the subset of genes in a cell that are active, and to what magnitude.
Bursts and splices
These methods provide a systematic way to study cells allowing us to understand the statistical aspects of the transcription process itself. Sandberg’s current work is looking at whether such transcription happens continuously in a conveyor-belt manner or in short, sharp bursts of activity. By being able to count RNAs in single cells and also new RNAs – made only in the last hours – they have found that transcription occurs in bursts with the magnitude and frequency of the bursts varying.
“We are now looking at how transcriptional bursting is dependent on specific biochemical regulators,” he said.
Sandberg also emphasised that current molecular analyses overlook an essential layer of complexity: alternative splicing, a mechanism that is essential for generating the diversity of proteins in our cell types. RNA variants in cells are often the consequence of alternative processing (or mis-splicing) and are involved in several neuropsychiatric diseases – like schizophrenia and autism. He explained that there is a high rate of splicing – “over 90% of the genes in our genomes are alternatively spliced at some point. From a public dataset of cells from the visual and motor cortex of mice brains, we can see that over 9000 genes show alternate splicing outcomes.”
“The single-cell revolution hasn’t quite reached splicing and I hope our laboratory will be able to change this, he said. “Our ongoing work is trying to comprehensively identify cell-type regulated alternative splicing in the brain. And one of our specific aims is to develop new computational techniques to identify disease-relevant, alternative-splicing changes that cause RNA variants. Integrating alternative splicing into single-cell analyses promises to fill a significant gap in our molecular comprehension of the brain’s functionality.”
Some of the issues the work hopes to address is cataloguing how much variation is tied to cell types in the brain and how to capture this variation; asking what the splicing code looks like in the brain and what the functional outcomes are; and, asking if spliced synaptic proteins explain neuronal electrophysiology and can predict different properties?
Sandberg also highlighted that substantial progress in deep-learning techniques and AI (including SpliceAI) –is making it much easier to predict where splice sites might occur in the sequence. “We are now performing transfer learning of splicing deep-learning models to distinguish cell-type specific splicing outcomes.”
He also pointed to the difficulties in sharing human genetic data and the tools that are becoming available to remove the more sensitive parts concerning genetic variation for more open data sharing.
“However, of course, we need to learn much more about how all this works before we use human models. There are new drugs and strategies that try to control splicing outcomes linked to disease but it isn’t common as yet. Our work is likely to be predictive, but we are far from any application as yet.”
Michelle Galloway: Part-time media officer at STIAS
Photograph: Noloyiso Mtembu